- NGI /
- Projects /
- WEATHER-MIC /
- WP2
WP 2 - The influence of weathering on MP aggregation and settling
The main tasks are to develop large-scale column experiments, characterize changes in microplastic aggregates and to provide a model to account for the influence of weathering and sedimentation.
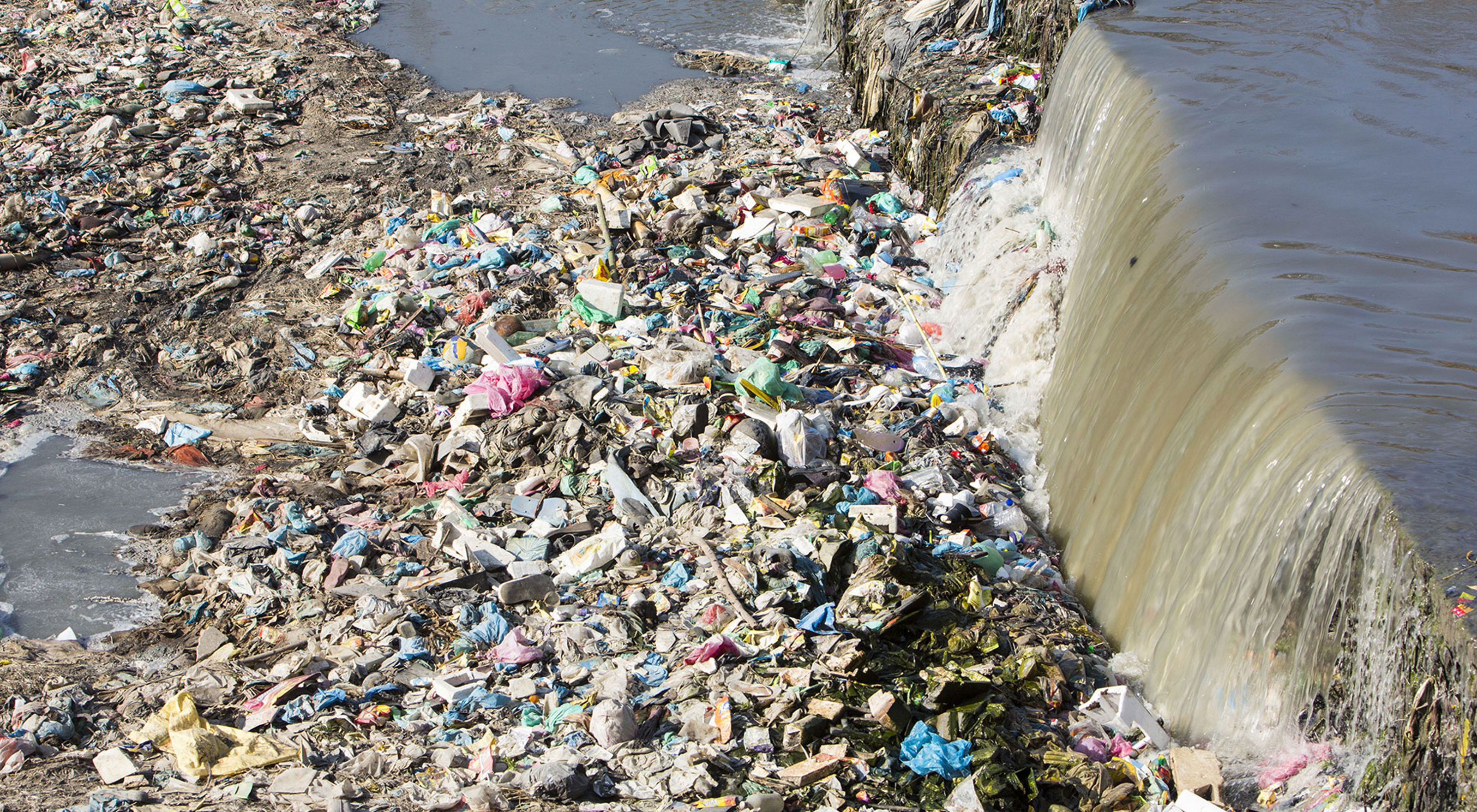
The objectives for WP 2 are to:
- develop a series of large-scale column experiments simulating weathering on diverse MPs in waters representative of the Baltic Sea (low salinity) and Oslo Harbour (mid to high salinity) under diverse conditions
- characterize the changes in MP-aggregates using diverse measurements (visualization, particle size distribution, density), that can be used to better account for field observations (WP5)
- provide a model to account for the influence of weathering and sedimentation, that can be implemented in a large-scale estuarine model (WP4)
Task 2.1. Development of large column aquarium experiments
In coordination with WP1, task 1, column tests (Figure WP2) will be performed with selected microplastics to test how weathering with UV light, physical abrasion, biofouling, and interaction with clays affect the vertical distribution and sedimentation of particles. Tests will be done with "fresh" plastics and aged plastics in WP1. Water conditions, including turbulence, will be representative of the ranges in the Oslo Harbor and Baltic Sea. These column tests will be done at NGI and IKTS for cross-validation, to check for reproducibility of selected parameters and reduce the time required to provide partners with sufficient amounts of weathered plastics.
Columns will be made of glass, ideally, 25 cm in diameter to minimize wall effects, and range in height from 30 to 50 cm. The base will contain a petri dish to collect settled particles. Shear turbulence will be adjusted by adding a propeller at a specified depth and speed (or stir bar), though secondary currents will be avoided. Plankton growth will be regulated through the use of commercially available plant growth lights in a room where that is the only light source; similarly, the UV light will be regulated by using commercial sterilization lights in aquariums.
Starting variables to be manipulated
(*** The number and increment of variables manipulated will depend on the time required for the experiments and the allotted person months):
- Type of MP (primary and secondary), done in collaboration with WP1
- Weathering: UV radiation, presence of biofilm, presence of charged clay materials (e.g. smectite) that may interact with charged colloidal plastics (<4 μm), leading to density changes
- Initial aqueous chemistry of the water (covering variation in salinity, DOC, and clay content similar to Stockholm water, and Oslo water)
- Laminar (no stirring) vs. turbulence (via the introduction of a stir rod)
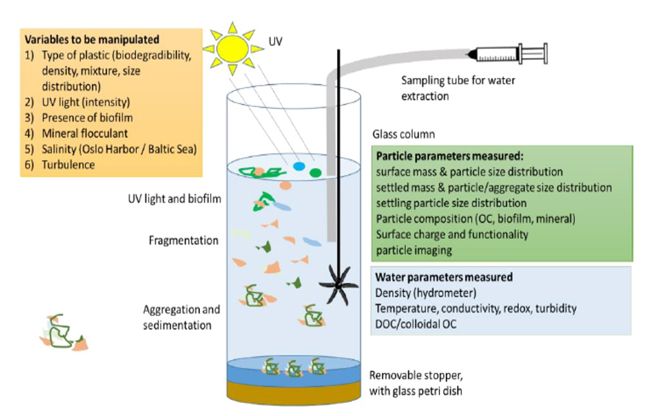
Figure WP2. Schematic for the column simulation (green = biofilm, other colors MP)
Water property parameters to be measured:
Density (hydrometer), temperature, conductivity, redox, turbidity, turbulence (Nortek Vectrino Profiler)
Particle properties to be measured (mostly as in WP1):
Changes in mass distribution and particle size distribution with depth will be measured to assess single-particle settling velocities ( ) and break-up and flocculation rates in still and turbulent water. This will be measured by monitoring changes in density, taking subsamples using the falling drop method, or simply by sieving/filtering different size fractions. For selected subsamples, particle size distribution and aggregation, as well as changes in morphology (non-sphericity) with depth will be measured by particle imaging analysis (Task 2.2).
Task 2.2. Particle imaging
Carefully selected subsamples will be removed from the columns via suction at the appropriate depth or removal of the petri dish after removing the overlying water. Samples from NGI for particle imaging will be freeze-dried after transferring them to glass filter papers, the edges of which are taped onto petri dishes. These are then tightly packed in a Styrofoam container and sent to IKTS.
The particle imaging analysis is part of WP1 (Task 1.4). For analyzing smaller particles on selected subsamples, scanning electron microscope (SEM) imaging will be applied. For particles produced at IKTS, a membrane filtration procedure (the filters have pore widths between 0,05 and 10 μm) could be used for sample preparation. With the techniques offered at IKTS we expect to significantly improve the analysis of particle size, form, and surface properties (charge, adsorption of dissolved organic matter).
The most important analysis is through dynamic imaging (QICPIC (Sympatec GmbH)), because we will be able to distinguish between primary particles and aggregates or agglomerates. One advantage of these devices is the concentration range, which can be characterized - even a very small number of particles in a large volume, as expected here, is detectable. Dynamic image analysis techniques are available and usable not only in the lab but also on cruises.
Disadvantages are the limitations of measurement range (not below 2 microns). For analyzing smaller particles on selected subsamples, scanning electron microscope (SEM) imaging will be done. For particles produced at IKTS, a membrane filtration procedure (the filters are between 0,05 and 10 μm pore width) could be used for sample preparation. Besides particle size, morphology, and form we will improve the analysis of particle surface properties (charge, adsorption of dissolved organic matrix).
Task 2.3. Mathematical modeling model of the column tests
Settling velocity rates ( ) measured through changes in mass and particle size distribution in different depths column experiment (measured by removing subsamples at different depths and at different times), and information on particle morphologies (e.g. non-sphericity) will be analyzed in terms of combined transport and reaction equations in time and the vertical space dimension.
To this end, the microplastic particle population is classified into different size ranges and different chemical types k = 1,…,N., with nk(z,t) being the number of particles belonging to class k per unit volume at height z and time t. For the sake of simplicity, we assume that all reactions between particles are 2 to 1 or 1 to 2, i.e., two particles coalesce into a single one or one particle breaks up into two. We designate the probability per unit time for a particle from class k to break up into two particles from classes i and j, respectively, by the break-up function → ( , γ̇, , . . . ).
It is reasonable to expect that the decay probability depends on temperature T, shear rate γ̇ and turbulence intensity K, but other factors like salinity might be important. The break-up rate is then given by → ( , γ̇, , . . . ) if nk is sufficiently small, which we will assume. In a similar way, we may express the flocculation rate for particles from classes j and k to class i as the product → ( , γ̇, , . . . ) .
Assuming turbulence to be homogeneous throughout the settling column and that there is no convection, break-up and flocculation aggregation diffusion and settling can be described mathematically by the Equation

The requirements for a consistent analysis of the column test data will to a large degree determine which experiments are needed to extract all relevant parameters:
- The single-particle settling velocities ( ) in still water needs to be determined for a collection of particles spanning the entire size and shape range under consideration
- The effective diffusion coefficient eff for particles depends mainly on turbulence, but may also depend on particle size and shape. Assuming a standard value for the Schmidt number, we can estimate Deff by measuring turbulence in the settling column (using a Nortek Vectrino Profiler) and the power input of the stirring apparatus
- Given the large number of parameters that need to be varied (e.g. salinity, turbulence, polymer type), the number of size classes will need to be reduced to a minimum. Recent research (Lee et al., Ocean Dynamics 64. (2014): 13-pp) suggests that three or even only two size classes (representing different populations) may give a satisfactory description for many practical applications. This issue will be investigated further in Task 4.3.
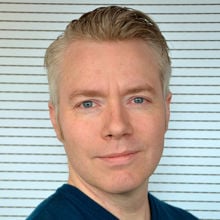